- Power Maxx Vibration Plate Manual Dexterity System
- Vibration Plate Soloflex
- Power Maxx Vibration Plate Manual Dexterity Test
Abstract
Taken from Wikepedia vibration white finger. Loss of manual dexterity (nerves and muscles affected): In more severe forms attacks may occur frequently in cold weather, not only at work, but during leisure activities, such as gardening, car washing or even watching outdoor sports and may last up to an hour causing considerable pain and loss of. For further inforamtion, about the Power Maxx Vibrations User Guide pdf file by clicking on this link: Power Maxx Vibrations User Guide No topics ever created yet. Power Maxx Vibration Plate Manual Lymphatic Drainage. Power maxx vibrations user guide: Download power maxx vibrations user guide. Enhance your fitness and health routine with this high-end foldable whole body vibration machine. This new machine is fully folding, has a built-in BMI Body Fat Analyzer and features high-ower 1500 watt dual motors.
Occupational exposure to vibration through the use of power- and pneumatic hand-tools results in cold-induced vasospasms, finger blanching, and alterations in sensorineural function. Collectively, these symptoms are referred to as hand-arm vibration syndrome (HAVS). Currently the International Standards Organization (ISO) standard ISO 5349-1 contains a frequency-weighting curve to help workers and employers predict the risk of developing HAVS with exposure to vibration of different frequencies. However, recent epidemiological and experimental evidence suggests that this curve under-represents the risk of injuries to the hands and fingers induced by exposure to vibration at higher frequencies (>100 Hz). To improve the curve, better exposure-response data need to be collected. The goal of this review is to summarize the results of animal and computational modeling studies that have examined the frequency-dependent effects of vibration, and discuss where additional research would be beneficial to fill these research gaps.
Introduction
Power Maxx Vibration Plate Manual Dexterity System
Workers that repeatedly use vibrating power- and pneumatic hand-tools are at risk of developing hand-arm vibration syndrome (HAVS). HAVS is characterized by cold-induced vasospasms in the fingers and hands that result in blanching of the skin, reductions in tactile sensitivity, pain, and changes in thermal thresholds. In addition, occupational exposure to hand-transmitted vibration may also contribute to reductions in grip strength and manual dexterity, muscle fatigue and atrophy and joint injuries, 2). In an attempt to protect workers from developing HAVS, the International Standards Organization (ISO) had developed a standard, ISO 5349-1, that contains a frequency-weighting curve to help workers and employers assess the risk of injury with exposure to vibration of different frequencies3). This curve assigns the greatest weight to lower frequency vibration exposures (i.e., <16 Hz) and significantly less weight to higher frequency (>100 Hz) exposures, indicating that lower frequency exposures generate a greater risk of injury than higher frequency exposures. However, epidemiological and experimental evidence suggests that the risk of injury, particularly to the fingers and hands, is greatest with exposure to vibration at higher frequencies (>100 Hz). Thus, although the current weighting curve may accurately estimate the risk of injury to the hand-arm system, it appears to underestimate the risk of injury to the fingers and hands associated with exposure to higher frequency vibration.
In order to improve the standard, better exposure-response data need to be collected. Collecting exposure-response data in humans is difficult; single exposure studies may not accurately represent what happens to workers who can be repeatedly exposed to vibration over years, and collecting these types of data from workers is difficult because they may use different vibrating hand-tools over time, and it is difficult to precisely determine what their actual exposure is. Therefore, both animal surrogate and computational models have been developed to predict how vibration induces injuries to the hands and fingers, and to examine the frequency-dependence of these injuries. The goal of this paper is to review the results of the animal and computational modeling literature that have examined the frequency-dependent effects of vibration and to determine what additional research needs to be performed to improve the ISO standard.
Animal Models
A summary of papers utilizing animal models to examine the effects of vibration on peripheral vascular and nerve, and skeletal muscle is presented in Table 1. These studies used primarily rats and exposed them to either hind limb or tail vibration. Most of these studies examined the effects of vibration at a single frequency (usually 30–60 Hz, accelerations of approximately 2–7 g). These studies have provided some information regarding the frequency-dependence of vibration-induced injuries and demonstrate that exposure to vibration at frequencies greater than 20 Hz induces changes in peripheral vascular and neural, and skeletal muscle structure and function. The tissue alterations seen in multi-day studies are consistent with changes seen in workers diagnosed with HAVS, but more detailed investigations are needed to elucidate exposure-response patterns and mechanisms of injury, .
Table 1
Summary of published research using animal models of vibration-induced injury
Frequencies | Exposure characteristics | Effects | Citation |
---|---|---|---|
45 Hz | Mouse 0.3 g acceleration 15 min/day for 6 wk whole body | reduction in number of capillaries, arterioles and venules in soleus muscle | 36) |
60 Hz | Rabbit 5 g acceleration 2 h/day for 75, 125, 225 or 300 days Hind limb | - myelin disruption and axon shrinkage - disruption of nodes of Ranvier - enlargement Schmidt-Lanterman incisures - axon cytoskeleton disruption | 37) |
50 Hz | Rat 1.8–7.1 g acceleration Single 3 h exposure Fore and hind limbs | - increased albumin in endothelium of capillaries. - indicative of increase in vascular permeability | 7) |
50 Hz | Rat 5 g acceleration 3 h/day for 60–90 days Fore and hind limbs | - increased vascular permeability between 60 and 90 days | 38) |
50 Hz | Rat 3.6 g acceleration 3 h/day for 90 days Fore and hind limbs | - increased vasoconstriction of peripheral arteries | 39) |
50 Hz | Rat 3.5 g acceleration 5 h/day for 2 or 5 days Hind limb vibration followed by sciatic nerve crush | - increased sensitivity to pinch test up to 1 month after nerve crush - indicative of peripheral nerve sensitization | 40) |
81 Hz | Rat 4.7 g acceleration 4 h/day for 2 days Hind limb | - increase in insulin-like growth factor staining in Schwann cells of sciatic and plantar nerves 2–3 days following exposure | 41) |
82 Hz | Rat 6.1 g acceleration 4 h/day for 5 days Hind limb | - edema in sciatic nerve | 42) |
82 Hz | Rat 6.1 g acceleration 4 h/day for 5 days Hind limb | - at electron microscopy level unmyelinated fibers in plantar nerve showed deranged axoplasmic structure - accumulation of endoplasmic reticulum - most recovered by 2 weeks following exposure | 43) |
60 Hz | Rat 5 g acceleration 4 h/day for 30 or 90 days Hind limb | - at 90 days disruption of internal elastic membranes in small arteries - thickening of intima and proliferation of vascular smooth muscle | 6) |
30, 60, 120, 240, 480 or 960 Hz | Rat 5.5 g acceleration Single 4 h exposure or 4 h/day for 14 days Hind limb | - increase in plasma creatine phosphokinase activity (CPK) with exposure to 30, 60, 120 and 240 Hz - increase greatest at 30 Hz - no morphological changes in skeletal muscle | 44) |
30, 60, 120, 240, 480 or 960 Hz | Rat 5.5 g acceleration Single 4 h exposure Tail and hind limb | - tail reduced temperature at 30 and 240 Hz - tail reduced blood flow at 30, 60 and 480 Hz - tail reduced nerve conduction velocity at 30, 60 and 120 Hz - hind limb vibration increase CPK at all but 960 Hz - hind limb vibration reduced brain norepinephrine levels in the hypothalamus at 120 Hz, increased dopamine levels in medulla oblongata and pons at 60 Hz and increased serotonin levels in hypothalamus at 120 Hz | 18) |
30 or 480 Hz | Rat 5 g acceleration 4 h/day for 30 days Hind limb | - disruption of the internal elastic membranes in small arteries at both frequencies - intimal thickening | 20) |
60 Hz | Rat 5 g acceleration 4 h/day for 1 or 9 days Tail | - 1 day of vibration increase in immunostaining for transcription factor NFATc3 in endothelial cells of ventral tail artery(VTA) - 9 days loss and thinning of endothelial cells, activated platelets in subendothelial tissue, evidence of constriction-induced injury in vascular smooth muscle and endothelial cells of VTA | 45) |
30, 60, 120 or 800 Hz | Rat 5 g acceleration Single exposure 4 h Tail | - increase in NFATc3 immunostaining in endothelial cells and vascular smooth muscle and increased vacuole formation at 30, 60 and 120 Hz in VTA - disruption of internal elastic membrane at 60, 120 and 800 Hz in VTA | 19) |
30, 120 or 800 Hz | 5 g acceleration single exposure 4 h Tail | - similar percentages of axons with disrupted myelin - intraneural edema and dilated arterioles | 24) |
44 Hz | rat 4 h/day for 7 days Anesthetized daily Hind quarters | - splitting in myelin sheath surrounding nerves - axon damage characterized by myelin balls and finger rings in myelinated and unmyelinated fibers | 46) |
60 Hz | 5 g acceleration 5 h/day for 2 or 10 days anesthetized daily Hind quarters | - reduced motor neuron axoplasmic transport - soleus muscle atrophy - progressive weakness hind limbs and difficulty walking | 47) |
40 Hz | Rat 5 g acceleration 5 h/day for 2 days Hind limb vibration followed by sciatic nerve dissection 7 days later | - hampered regeneration of the sciatic nerve 6 and 8 days after dissection | 48) |
125 Hz | Rat 5 g acceleration Single 4 h exposure Tail | - increased sensitivity of VTA to α2C-adrenoreceptor-mediated vasoconstriction 1 h after exposure - histological evidence of vasoconstriction | 49) |
125 Hz | Rat 5 g acceleration Single 4 h exposure Tail | - temporary increase in sensitivity to transcutaneous electrical stimulation of Aβ nerve fiber in tail | 50) |
125 Hz | Rat 5 g acceleration Single 4 h exposure Fore limbs | - reduced responsiveness of digital arteries to α-1 adrenoreceptor-mediated vasoconstriction - increase in the generation of reactive oxygen species | 17) |
125 Hz | Rat 5 g acceleration 4 h/day for 10 days Lean and obese Zucke r tail | - vibration induced an increase in vascular hydrogen peroxide levels and reduced sensitivity to acetylcholine-induced vasodilation in all rats - obese rats showed a reduction in tail temperature in response to vibration - lean rats displayed an increased sensitivity to α2C-adrenoreceptor-mediated vasoconstriction | 51) |
62.5, 125 or 250 Hz | Rat 5 g acceleration 4 h/day for 10 days tail | - reduction in internal diameter of VTA at 250 Hz - increase in thickness of vascular smooth muscle wall in VTA at 250 Hz - increase in reactive oxygen species at 250 Hz | 15) |
Biodynamic response of the tail to sinusoidal vibration
Vibration Plate Soloflex
Experimental studies have demonstrated that the physical (i.e., biodynamic) response of the human hand-arm system is frequency dependent, . The resonant frequency (i.e., the frequency or frequencies at which the oscillation of the tissues are amplified) of the hand-arm system as a complete unit is between 16 and 62.5 Hz. However, the resonant frequency of individual fingers is in the range of 150–300 Hz, and vibration at frequencies greater than 100 Hz is only transmitted to the tissues of the fingers and hands, and is not transmitted to the rest of the hand-arm system, . Absorption of these higher frequency vibrations by the tissues of the fingers and hands may result in greater shear stress and strain in the local soft tissues, and it has been hypothesized that the increase in tissue stress and strain induced by these exposures may increase the risk of injury. Therefore, understanding the biodynamic response of the tissues to different vibration frequencies is critical for understanding how frequency may affect the risk of injury.
To test this hypothesis, a NIOSH research team modified the existing rat tail models of vibration-induced injury and assessed vibration transmissibility to the tail to determine how it compared to transmissibility of the human fingers. A diagram of the model can be seen in Fig. 1. Rats are confined in Broome style restrainers, and their tails are secured to a platform with elastic straps. A laser vibrometer measured vibration transmissibility to the tail at 1/3rd octave band frequencies between 31 and 500 Hz, and at constant accelerations of 1, 5 and 10 g. The graphs in Fig. 2 show vibration transmissibility to the tail is frequency- and location-dependent. At frequencies at or below 62.5 Hz, transmissibility is near 1, meaning that the tail is moving in unison with the platform. However, between 125 and 250 Hz, transmissibility significantly increases, meaning that the vibration is actually being amplified in the tail. The peak amplification, or resonant (natural) frequency, of the mid-portion of the tail is between 125 and 300 Hz. The resonant frequency is slightly shifted depending upon the acceleration used and the precise location of the measurement, but all animals tested displayed resonant frequencies in the same range. Human fingers also display a range of resonant frequencies (i.e., 125–300 Hz), depending on the location of the measurement and grip force used when the measurement was taken. The peak magnitude of the resonant frequency of the fingers isn’t as great in the fingers as it is in the tail, primarily because the mass and stiffness of the tail are less than those of the fingers. However because the frequency-dependent response of the fingers and tail are similar within this range, the tail can serve as a model for understanding the exposure-response relationship between vibration and tissue injury.
A diagram of the rat tail model and apparatus used to measure vibration transmissibility.
Photograph of the tail identifying the location of transmissibility measurements. The graphs show transmissibility data over the length of the tail at three representative frequencies.
The frequency-dependent responses of vibration on soft tissues
Peripheral vascular morphology and function
A study was conducted using the NIOSH rat tail model of vibration-induced injury to assess the effects of frequency on peripheral vascular and nerve dysfunction. Rats had their tails exposed to vibration for 4 h/day for 10 days at 62.5, 125 or 250 Hz with a constant acceleration of 5 g (49 m/s2 rms). Ventral tail arteries and nerves were collected from the mid-portion of the tail 24 h following the last exposure. This region of the tail was selected because the biodynamic response to vibration was different at these three frequencies, and thus by examining tissue in this region the effects of frequency could be assessed (Fig. 2).
Figure 3 shows the effects of vibration on morphology of the ventral tail artery. Vibration at 250 Hz resulted in a reduction in the size of the lumen and an increase in the thickness of the vascular smooth muscle (media layer), indicating that after exposure to vibration at 250 Hz the arteries were remodeled and narrowed. These changes in morphology were accompanied by an increase in immunostaining for nitrotyrosine, a marker of increased oxidative activity, and interleukin-6 (IL6), a factor involved in remodeling. Increases in oxidative stress and vascular remodeling often precede vascular dysfunction, . Both the unweighted and ISO weighted accelerations are displayed on the x-axis of these figures. Based on the weighted accelerations, changes in vascular morphology should have been greatest at 62.5 Hz. But instead, vascular changes were greatest at 250 Hz, the resonant frequency in this region of the tail. These data are consistent with the hypothesis that frequencies that cause the greatest stress and strain on tissues will have the greatest effects. This does not mean other frequencies will not affect vascular function or morphology. However, these data suggest that the changes may occur more quickly or be more dramatic with exposure to vibration at or near the resonant frequency.
Photomicrographs of the ventral tail artery from rats exposed to restraint-control conditions or vibration for 10 days. The graphs show the (A) internal diameter and (B) thickness of the muscle walls from arteries collected from the rats in the various treatments. The x-axis displays frequency of the vibration along with the unweighted acceleration (U) and the ISO-weighted acceleration (W). The internal diameter of arteries was smaller in rats exposed to vibration at 250 Hz than in cage- or restraint-control rats (A: * less than cage or restraint controls, p<0.05). Vibration at 250 Hz also resulted in an increase in the thickness of the vascular smooth muscle (# greater than cage control, p<0.05). Data are expressed as group means ± sem.
These results indicate that there is time- and frequency-dependent adaptation of peripheral blood vessels to vibration because unlike the frequency effects noted at 10 d, Okada reported that a single exposure had an opposite response; the greatest reduction in tail blood flow was seen at low frequencies (i.e., 30 Hz, constant acceleration of 5 g). Other single day exposure studies examining the frequency-dependent effects of vibration on vascular function have shown that exposure of the tail to vibration between 30 and 800 Hz can cause morphological disruption of endothelial and smooth muscle cells and function in arteries–. In the rat tail artery, vibration exposure for 9, 10 or 28 d, reduced the lumen diameter by vasoconstriction suggesting that there was structural and functional adaptation of the media smooth muscle layer. In the hind limb, intimal thickening and lumen obstruction in the arteries was reported for both 30 and 480 Hz after 30 d of exposure. Unfortunately the magnitude of this pathology is unclear because there was not quantification of the occurrence, .
The different responses in these studies may be due to location specific vascular responses (i.e., tail versus hind limb). In studies utilizing the tail, the duration of the vibration differed and could account for the various outcomes. In addition, the methods for securing the tail to the platform, the location used for sample collection, and the length of the exposure were varied. The frequency-dependent biodynamic response of the tails and hind limbs to vibration in these studies is also unknown. What all these studies suggest, however, is that exposure to vibration frequencies over a wide range (30–800 Hz) can have detrimental effects on peripheral blood vessels. These findings are consistent with other research suggesting that the frequency-weighting curve may underestimate the risk of injury associated with exposure to frequencies > 100 Hz. There is a clear need for temporal examination because the frequency response of the naïve artery after a single exposure to vibration is not necessarily predictive of adapting arteries exposed for multiple days. Exposure to multiple and mixed frequencies should also be examined because workers developing HAVS are exposed to years of vibration at multiple frequencies.
Peripheral nerve morphology and function
The NIOSH study also measured frequency-dependent changes in peripheral nerve function using transcutaneous electrical stimulation. The tails were stimulated at 2 kHz with a transcutaneous electrical signal that assesses the function of large myelinated (i.e., Aβ) fibers. These fibers carry information from mechanoreceptors in the periphery to the central nervous system. Rats were tested prior to vibration exposures on the first day of the study and on day 9, prior to vibration exposure. Exposure to vibration at 62.5, 125 and 250 Hz resulted in a reduction in the perception threshold (i.e., an increase in sensitivity; Fig. 4). Increases in the sensitivity of nerves to various forms of stimulation are often a sign of injury. If nerve injury results in degeneration and loss of myelinated and unmyelinated axons the thresholds will increase. Okada et al., found that immediately following exposure to vibration at 30, 60 or 120 Hz, nerve conduction velocity was reduced, whereas there was not a significant reduction at 240, 480 or 960 Hz. These studies suggest that nerve function may be more sensitive, or have different frequency-dependent responses to vibration than arteries. The difference in the responses between these tissues may be due to delayed effects in the nerves or the difference between single versus multiple exposures.
Mean (± SEM) thresholds to transcutaneous electrical stimulation collected prior to vibration exposure on days 1 and 9 of a 10 day exposure regime (i.e. current perception threshold or CPT at 2000 Hz which primarily assesses the sensitivity of the large myelinated Aβ nerve fibers to stimulation). The x-axis shows the exposure frequency and the unweighted (U) and ISO-weighted (W) accelerations. On day 9 of the study, all rats exposed to vibration displayed a reduction in the CPT in comparison to controls, and day 1 measures. Data are expressed as the mean ± SEM CPT, * p<0.05).
Morphology of ventral tail nerves from control- and vibrated-rats also were analyzed in a number of studies, 24). In the NIOSH study, there was no change in the number of myelinated axons within the nerves, but there was a reduction in myelin thickness at 250 Hz at 10 days (Fig. 5A). There was also an increase in albumin staining in nerves collected from rats exposed to vibration at 250 Hz, which is indicative of vascular leaking and neural edema (Figure 5B). Govindaraju et al.24), found that a single exposure to tail vibration at 30, 120 or 800 Hz resulted in swollen and disrupted myelin, intraneural edema and dilated arterioles within the ventral tail nerve. The myelin damage and thickening observed immediately after a single 4 h vibration exposure, and 24 h post-vibration suggests that the reduction in myelin thickness after 10 days represents earlier demyelination/Schwann cell injury followed by remyelination, 24, . Reductions in myelin thickness may allow an electrical signal to spread from axon to axon more quickly, thereby reducing thresholds for stimulation. However, changes in sensitivity to transcutaneous electrical stimulation were seen at all three vibration frequencies. These changes in sensitivity may have been the result of vibration-induced changes at the level of the sensory receptors, changes in ion channel numbers, or some other adaptive change in nerve physiology.
Myelin thickness (A) and the area stained for albumin (B: % of controls) in the ventral tail nerves of rats exposed to vibration for 10 d. Exposure to vibration at 250 Hz resulted in a decrease in myelin thickness and an increase in albumin staining.
Impact vibration
Impulsive or percussive tools have fundamental frequencies in the 20–70 Hz range, generated by the impact or duty cycle. With each impact, a brief shock wave, msec in duration, is generated. The shock wave contains substantial power in the kHz frequency range. Average unweighted accelerations of 100 m/s2 and peak accelerations ≥ 20,000 m/s2 are common for percussive tools. Shock waves are less abundant and of lower magnitude in rotary tools. ISO 5349 frequency weighting minimizes the energy contribution in the higher frequency range (kHz), reducing accelerations to 7–20 m/s2 for impact tools. Unfortunately, the prevalence of neural and vascular symptoms occur at higher percentages (risk) than predicted from ISO 5349, . This discrepancy suggests that the high accelerations in the high frequencies maybe harmful and thus, should not be excluded from risk calculation by frequency weighting.
Studies of the tissue injury from impact vibration tools are surprisingly lacking. Recently, Govinda Raju et al. developed a rat tail model to assess the effects of impact vibration generated using a riveting gun. A single 12 min exposure produced immediate damage to nerve endings in the skin, mast cell degranulation and hypersensitivity to thermal stimulation. Four days later, the skin became hyposensitive to thermal stimulation, and the number of PGP 9.5 (a marker used to identify nerves) immunolabeled nerve endings had decreased (Fig. 6). Reduced thermal sensitivity and loss of feeling in the fingers are characteristic of established HAVS. Further animal studies are necessary to examine injury of the other soft tissues (e.g. artery, muscle, skin) and ascertain whether the injury is attributable to specific frequencies, especially in the kHz range, or the very high accelerations generated by percussive tools. Sinusoidal tail vibration experiments have shown that 60 Hz causes arterial injury in a 2-step process consisting of vibration-mediated vasoconstriction with smooth muscle cell blebbing and acceleration shearing off the blebs24). The blebs are large projections from the cells connected by slender stalks that are presumably readily broken by acceleration stress and strain. The rat tail impact vibration model has demonstrated rapid and severe tissue injury. These findings emphasize the need for an ISO regulation that considers the contribution of vibration energy from high frequencies when assessing risk to workers using percussive tools.
Immunostaining the rat tail skin with PGP9.5 antibodies reveals intact nerve endings in the sham control. Four days after a single 12 min exposure of the tail to riveting hammer impact vibration, fragmented destruction of the skin nerve endings is present. Arrows point to PGP9.5 immunostained nerves. Bar equals 35 μm.
Limitations of animal models
In humans, frequency and other factors such as the direction, the amplitude, the duration, the temperature, and the working posture and grip force on the tool contribute to the risk of developing HAVS. Animal studies can be used to examine the effects of vibration frequency, amplitude and temperature on the risk of developing a vibration-induced injury. The effects of duration of the exposure (both daily and cumulative) could be examined in animals. However, because it can take humans years to develop the symptoms of HAVS, it isn’t practical to attempt to replicate cumulative human duration exposures using animals. Animal models also cannot be used to examine the effects of posture, grip or force. In addition, animals are often restrained during exposures, and although restraint-induced stress and immobilization can contribute to changes in vascular and sensorineural responses–, most studies control for these effects. However, even with these limitations, animal models do provide evidence that exposure to vibration at frequencies greater than 100 Hz pose a significant risk for injury to vascular and peripheral nervous system tissues. They also provide direct evidence linking the biodynamic response of tissue to injury and, therefore, provide a framework for understanding how frequency may affect the risk of injury in workers.
Computational models
Computational models of the fingers can be used to predict how factors contributing to HAVS, such as vibration frequency and amplitude, and applied force, contribute to the risk of injury. Both 2 dimensional (2D) and 3D finite element models have been developed to examine the responses of the finger to vibration, . These models include the bone of the finger, the nail and use viscoelastic properties to define the soft tissues. These models predict that the resonant frequency of the finger is in the range of 125–300 Hz depending upon the amplitude and applied force used in the simulated exposure (Fig. 7). These findings are consistent with experimental studies that measure the frequency-dependent biodynamic responses of the fingers-hand to vibration. These studies also predict that tissue stress and strain is absorbed locally by the soft tissues at the resonant frequency, and that the absorption of vibration by these tissues induces high levels of stress and strain. Thus, the results obtained using mathematical and animal models are consistent with the hypothesis that exposure vibration frequencies that induce the greatest tissue stress and strain could induce changes in vascular and sensorineural function that morphology that lead to the development of HAVS.
Finite element simulations of the vibration effects on the soft tissues at the fingertip. A: The finite element fingertip model of the soft tissues, bone, nail, and vibration plate. B: The simulations show the frequency-dependent responses of the fingertip. The vibration penetrations into the soft tissue reached a maximum around 250–500 Hz; at very high frequencies (>1,000 Hz), the vibration concentrated only on the skin surface and did not penetrate deep into the soft tissues. As indicated by the key, red represents the greatest level of penetration into tissues and blue represents the lowest level of penetration.
Limitations of computational models
Modeling studies provide predictions about the potential tissue stress and strain endured by soft tissues during exposure to vibration. Computational models cannot account for postures, repetitive exposures, or the adaptive responses tissues can display in response to mechanical exposures. Current modeling, albeit state-of-the-art, is limited to the tissue level and, because of the enormous complexity, does not model the cellular and subcellular level of resonances and injury susceptibility. However, the results of the modeling studies taken with those of the animals studies provide strong evidence that vibration induces the greatest stress to tissues of the fingers at or near the resonant frequency and that these stresses and strains are associated with an increased risk of injury.
Summary and future directions
Studies performed using animal and computational models are consistent with human studies demonstrating that exposure to vibration frequencies between 100–300 Hz results in an increase in the biodynamic response of the fingers and demonstrate that there is a link between increased tissue stress and strain and the development of vascular and sensorineural dysfunction. These data also support epidemiological and experimental work demonstrating that the exposure to vibration at frequencies greater than 100 Hz may increase the risk of vascular and sensorineural dysfunction in the fingers and hands of workers exposed to these higher frequencies, and that the frequency weighting curve presented in the ISO 5349-1 standard may underestimate the risk of vibration-induced injuries to the systems at these higher frequencies, . Additional studies examining the effects of other work- related and personal factors that can contribute to the risk of developing HAVS also would provide valuable information that could be used to improve the current standard, and to provide information to guide tool makers and manufacturers of personal protective equipment toward more effective designs that lower vibration exposure in the workplace.
Acknowledgments
Funded in part by NIOSH grant R01 OH003493 to DAR.
References

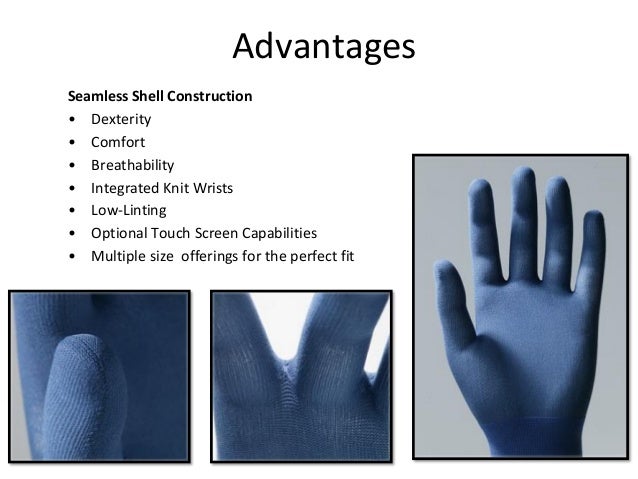
Power Maxx Vibration Plate Manual Dexterity Test
'Vibration White Finger is nothing to fool around with and youshould see your doctor immediately. Some of the symptoms to watchfor are your fingernail, and rest of your finger from the lastknuckle down turning white and going numb.'
What are the causes and symptoms of vibration white finger?
'The causes of vibration white finger are the continual use of heavy machinery and can become a permanent disease. Some symptoms of the disease are numbness in the fingers, tingling sensations, coldness and the feeling of pins and needles.'
What syndrome is 'vibration white finger' generally associated with?
Vibration white finger is a work injury in that one's hand or fingers become white, numb, and one loses finger mobility. It is caused by the fingers and hands vibrating too much as with using a jackhammer.
What are symptoms of mallet finger?
Symptoms of mallet finger include pain and swelling around the top part of the finger, near the outermost joint.
Symptoms of arsenic poisoning?
Arsenic poisoning is characterized by high levels of arsenic in a person's body. Symptoms include headache, nausea, diarrhea and white lines in the finger nails.
What are the Symptoms of a out of balance tire?
What symptoms might occur with finger pain?
There are many symptoms that might occur with finger pain. Some of these symptoms include throbbing, soreness, tingling, stiffness or increased warmth.
What should a patient do if he has symptoms of mallet finger?
If symptoms of mallet finger appear, the affected individual should consult a physician or seek emergency care.
Symptoms of a bad flex plate?
Vibration, Clunking noise, starter not working correctly.
How do you get white knuckle disease?
This is secondary form of Raynaud's disease, from the continuous use of vibrating hand-held machinery. You get white knuckles from the damage to the blood vessels in your hands. You can also develop numbness in your fingers (nerve damage) and loss of manual dexterity(muscle damage). This is a cumulative disease and does not happen overnight. You need to regulate your 'trigger time', the amount of time you operate using handheld devices. Regardless if you use…
What are the symptoms of trigger finger?
pain in the fingers and 'popping' sensations. Sometimes the finger may lock down into the palm or lock out straight. Symptoms are usually worse in the morning and improve during the day.
On what did Snow White prick her finger?
Snow White does not prick her finger. Out of all the Disney Princesses, only Sleeping beauty (Aurora) pricks her finger.
When do symptoms from mallet finger appear?
These symptoms occur right after the injury. Redness and swelling develop soon afterward.
Why is the tip of your finger nail white?
The tip of your and my finger nail is white because the tip of you finger dose not get blood and the rest dose so only the tip turns white. Marenda Baker
Why is your finger white after being in the cold?
Your finger turns the colour white after being in the cold because the blood circulation in your finger slows down to warm up the rest of your body. hence the white color.
If you finger a girl there is white stuff on your finger when you pull out what is that?
Signs and Symptoms of a jammed finger?
What are the signs and symptoms of a sprained finger?
The signs and symptoms of a sprained finger include: Pain and tenderness at the affected joint Pain when bending or stressing the finger Swelling and restricted mobility of the finger Instability of the finger in case of severe injury or complete ligamental rupture. First aid typically consists of rest, ice (single ice cube rubbed on joint works well) and use of a commercial finger splint, tongue depresser, or taping of two fingers together to provide…
What are the symptoms of the Wolff Parkinson White Syndrome?
There are many symptoms of Wolff-Parkinson-White Syndrome. Some of these symptoms are heart palpitations, fatigue, lightheadedness, anxiety and chest pain.
When is surgery necessary for trigger finger?
In severe cases that do not respond to injections and the finger or thumb remains in a locked position, surgery may be required to relieve the symptoms
Why do I have white finger while I was using hydrogen peroxide?
Simple your finger is infected.. nothing serious.
What are the symptoms of behavioral anxiety?
Behavioral symptoms of anxiety include pacing, trembling, general restlessness, hyperventilation, pressured speech, hand wringing, or finger tapping.
What causes the circulation in the finger tips to stop and fingers to become numb?
Loss of Circulation in Fingertips Raynaud's phenomenon (RP), also known as Red, White and Blue disease- is a condition resulting in a series of discolorations of the fingers and/or the toes after exposure to changes in temperature (cold or hot) or even emotional events ie.. anxious at the dentist or at a job interview. It is due an abnormal spasm of the blood vessels which results in a diminished blood supply to the local tissues…
What happens if you have a white dot on your finger?
If a pinky knuckle is broken what are the symptoms?
I'm pretty sure there are no symptoms. You will know if your pinky is broken IF....There is no swelling, no pain, and it works normally. Those are clear signs of a broken finger.
What do chillblains look like?
Symptoms Ulceration of the digits and toes Symptoms Red nose Skin redness Toe skin inflammation Finger skin inflammation Earlobe inflammation
What is the vibration of a tuning fork?
if u hit it on a wooden desk (like a classroom desk) it will vibrate and you can see and feel the vibration of a tuning fork like that. If u touch it ur finger/hands will keep it from vibrating . ur welcum 4 telling u d answer! 'peace!'
Why sound is produced when you break your fingers?
If any sound is produced, it would be due to the vibration of the different materials(bone,tissue, cartilage etc) that composes the finger as it breaks.
What are symptoms that teens may complain?
Teens can get many symptoms from not using proper ergonomics. They start to complain of neck and shoulder pains. They also start to get neck cramps and finger pain.
What are the symptoms of a werwolf?
1) your third finger is longer than your middle finger 2) you have hair growing on your palms an d inside your stomach b careful dont be a werewolf ur self!!
How do harps make sound?
Sound on the harp is produced by plucking the strings. The finger plucks the string, setting it into vibration. This wave of vibration travels down the string to the wooden soundboard where it is amplified (or made louder). On Irish harps the fingernails are used to pluck. On large 'pedal' harps or 'concert' harps, the pad of the finger is usually used. However, there are examples of using nearly everything; from the knuckles and palms…
What STD can cause white spots on your tongue?
White spots on the tongue is one of the symptoms of HIV. Rapid weight loss and a dry cough are also HIV symptoms.
What are the white spots underneath your fingernails?
The white spots underneath your fingernails are something like eternal bleeding. It would be fungi growing underneath your finger, because you had hit your finger against something. For example, if you squeeze your finger in between a door the skin under neath your finger might rip causing fungi to grow from all of the bacteria.
Why do you have white in your finger nails?
it is a condition called leukonychia not seareous its only cuz you engerd your finger nail in the past cupple of weeks
Types of vibration?
mainly two types of Vibration measurement: shaft vibration Bearing Vibration
My front brake calipers getting hot on your 99 gmc Yukon?
Brakes do get HOT ~400 degrees actually. What is the symptoms your are experiencing, fading, brake smell, vibration?
What are symptoms of a faulty driveshaft?
A vibration felt throughout the vehicle that gets worse with speed, a rotating squeak noise, a 'clink' noise when shifting between drive and reverse.
How can you tell if a tendon has been cut inside your pinkie finger?
You would be unable to bend or lift your finger if the tendon is cut completely. However, you would also have an indication of this (along with the above symptoms) because it would mean your finger has been deeply cut in a severe injury.
What are the symptoms of tardive dyskinesia?
Some symptoms of tardive dyskinesia are facial grimacing, finger movement, jaw swinging, repetitive chewing and tongue thrusting. Also delayed abnormal movement in the lower face.
What are lunules?
Lunules are the small white areas at the bottom of the finger nails
When a guy puts his finger in your vagina why do you get white secretion?
What is a small cluster of spot pimple-like bumps just under the skin surface on your finger that release a clear liquid when squeezed?
im not a doctor, but the clear stuff is probably white blood cells. You might Try researching Dyshidrosis, it sounds similar to your symptoms
Symptoms of psoriasis?
Is white liquid fluid coming after vagina symptoms of pregnancy?
What are the symptoms of touching a white tree frog?
What is treatment for trigger finger?
Initial treatment for mild or infrequent symptoms of trigger finger include rest, avoiding or modifying those activities that caused the inflammation, and the use of a nonsteroidal anti-inflammatory drug (NSAID) such as ibuprofen
Why do humans get white cloud-like things on your nails?
Because they are your finger nails and if you press them then they will become white in colour.
What is the reason for white under the fingernails?
One reason for white under the finger nails is zinc deficiency, a.k.a. hypozincemia.
You put your finger in your vagina too check to see if everythings ok and you pull your finger out and on your finger will be this white stuff that looks like snot everyone says its cum but you dont g?
no its not it discharge and its natural for a girl to get that
Can you make a sentence with the word vibration?
Here are some sentences. She felt the vibration of her cellphone in her pocket. The vibration of the sound waves allows us to hear noise.
Do males get symptoms if they have gonorrhea?
From the related link, CDC: 'Some men with gonorrhea may have no symptoms at all. However, some men have signs or symptoms that appear two to five days after infection; symptoms can take as long as 30 days to appear. Symptoms and signs include a burning sensation when urinating, or a white, yellow, or green discharge from the penis. Sometimes men with gonorrhea get painful or swollen testicles.'. Yes, men often do show symptoms when…